Immune Checkpoint Blockade: InestimAble Advances
Over the last decade, the understanding of key steps in the regulation of T cell responses has led to the groundbreaking development of immune checkpoint blocking monoclonal antibodies (mAbs) to fight cancer. The first FDA-approved mAbs have provided unprecedented remissions in melanoma and non-small cell lung cancer, although with considerable variation in response rates (10% to 90%) and significant toxicity1,2. This revolution in cancer therapy is now the basis for new IC-based curative strategies.
T-cell responses first rely on the T-cell receptor (TCR) recognition of MHC:peptide complexes on antigen presenting cells (APCs). Further engagement of co-stimulatory and co-inhibitory molecules guarantees the onset and the limitation of T-cell activities. These molecules have rightfully been named “immune checkpoints” (ICs). Initial studies focused on relieving the immunosuppresive brake by the co-inhibitory CTLA-4 (cytotoxic T lymphocyte associated protein 4) and PD-1 (programmed cell death 1) receptors3. CTLA-4 is expressed by activated and regulatory T cells (Tregs), and exerts competitive binding for stimulatory CD28 ligands (CD80/CD86). PD-1 is expressed by activated and exhausted T cells, and binding to its ligands PD-L1 and PDL-2 directly inhibits TCR signaling through SHP2-mediated dephosphorylation of proximal signaling elements3. Of note, recent findings point CD28 as a convergent regulation target for both CTLA-4 and PD-1, and call attention to the regulation of intra-tumoral T-cell trafficking by PD-13.
Therapeutic IC-blocking mAbs have a dual activity inherent to their structure: while the variable regions bind to IC-epitopes, the “fragment crystallizable” (Fc) mediates targeted cell death through selective interaction with the complement molecule C1q (CDC: complement dependent cytotoxicity) and the Fc receptors on innate effector cells (ADCC: antibody dependent cellular cytotoxicity, ADCP: antibody dependent cellular phagocytosis)4. To date, approved IC-mAbs are IgG1s and IgG4s depending on the necessity to protect or kill the target cells5. Anti-CTLA4 ipilimumab and anti-PDL1 atezolizumab are IgG1s that are expected to cause preferential Treg and tumor cell depletion, respectively1,3. On the contrary, anti-PD1 nivolumab & pembrolizumab are modified IgG4s with low effector functions, mainly operating through blocking PD-1 interaction with its ligands1,5. Altogether, these IC-mAbs allow better activation of effector T cells, and the combination of anti-CTLA4 and anti-PD1/PDL1 improves survival1,3.
The challenge of IC immunotherapy is to improve the mAb response rates in a larger panel of cancers. A first approach is to modulate mAbs’ functionality through Fc engineering6 (see next page). For example, atezolizumab is a non-glycosylated IgG1 that retains its blocking activity but lacks cytotoxic functions3. New anti-CLTA4 mAbs with increased or dampened effector functions have also been developed and are under clinical trials1. Other strategies targeting more co-inhibitory molecules on T cells (e.g. LAG3, TIM-3, TIGIT, VISTA) have entered clinical trials, even though their biological roles is not fully understood3,7. The next generation of therapeutic mAbs also includes agonist agents targeting co-stimulatory molecules (OX40, ICOS, GITR, 4-1BB, CD40) on T cells to potentiate effector responses3,7. Importantly, combination of the above-mentioned approaches hold important promises7.
The future of anti-tumor immunotherapy relies on the induction of responses at multiple levels, including harnessing of other effector cells (e.g. natural killers, neutrophils) in addition to T cells. As an example, anti-NKG2A monalizumab blocks inhibitory signaling in natural killer cells and subsets of cytotoxic T cells, and further potentiates other therapeutic mAbs8. The identification of reliable predictive biomarkers and the combination of IC-therapies with other immunotherapies (e.g. adoptive T cell-therapy, oncolytic viruses, agonists for pattern recognition receptors), and radio/chemotherapies, may lead to the ultimate maximization of response rates9.
References:
1. Kavecansky J and Pavlick A.C. 2017. Beyond checkpoint inhibitors: the next generation of immunotherapy in oncology. AJHO. 13(2):9.
2. Ribas A. and Wolchock J.D. 2018. Cancer immunotherapy using checkpoint blockade. Science. 359:1350.
3. Wei, S.C. et al. 2018. Fundamental mechanisms of immune checkpoint blockade therapy. Cancer Discov. 8(9):1069.
4. Quast I. et al. 2017. Regulation af antibody effector functions through IgG Fc N-glycosylation. Cell. Mol. Life. Sci. 74(5):837.
5. Almagro, J.C. et al. 2018. Progress and challenges in the design and clinical development of antibodies for cancer therapy. Front. Immunol. 8:1751.
6. Whang, X. et al. 2018. IgG Fc engineering to modulate antibody effector functions. Protein Cell. 9:63.
7. Donini, C. et al. 2018. Next generation immune-checkpoints for cancer therapy. J Thorac Dis. 10 (suppl 13): S1581.
8. André P. et al. 2018. Anti-NKG2A mAb is a checkpoint inhibitor that promotes anti-tumor immunity by unleashing both T and NK cells. Cell. 175:1731.
9. Marshall H.T. and Djamgoz B.A. 2018. Immuno-oncology: emerging targets and combination therapies. Front. Oncol. 8:315.
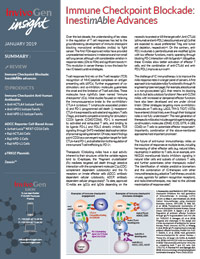
InvivoGen's Insight Newsletter - January 2019
Immune Checkpoint Anti-human Antibodies
ADCC Reporter Cell-Based Assay
- Jurkat-Lucia™ NFAT-CD16 cells
- Raji-hCTLA4 Cells
- Raji-hPD-1 Cells
- Raji-hPD-L1 Cells
- pTRIOZ plasmids
- Zeocin® selective antibiotic
Download our latest Insight Newsletter (January 2019).